Equations in thermodynamics play a pivotal role in understanding the laws governing energy transfer and transformation. These mathematical expressions enable scientists and engineers to predict how systems respond under various conditions, guiding the design of everything from engines to refrigerators. By analyzing these equations, we can uncover the principles that dictate the behavior of matter and energy, forming the backbone of modern thermodynamic theory.
As we delve into the world of thermodynamics, it becomes clear that equations are not just abstract concepts; they are essential tools that allow us to quantify and manipulate the interactions between heat, work, and internal energy. From the fundamental laws to the various applications, these equations serve as a language through which we can communicate complex ideas in a structured and comprehensible manner. Whether you are a student, a researcher, or a professional in the field, understanding these equations is crucial for applying thermodynamic principles effectively.
In this article, we will explore the various equations in thermodynamics, their significance, and how they apply to real-world scenarios. We will address common questions that arise in the study of thermodynamics and provide insights into the mathematical relationships that govern energy systems. Join us on this enlightening journey as we unravel the complexities of thermodynamic equations and their applications in science and engineering.
What Are the Fundamental Laws of Thermodynamics?
The foundation of thermodynamics rests on four fundamental laws that govern the behavior of energy. These laws are encapsulated in equations that describe how energy is conserved, transformed, and transferred in a system. The laws are as follows:
- Zeroth Law: If two systems are each in thermal equilibrium with a third system, they are in thermal equilibrium with each other.
- First Law (Law of Energy Conservation): Energy cannot be created or destroyed; it can only be transformed from one form to another. This law is often expressed with the equation ΔU = Q - W, where ΔU is the change in internal energy, Q is the heat added to the system, and W is the work done by the system.
- Second Law: The total entropy of an isolated system can never decrease over time. This law is often illustrated through the equation ΔS ≥ Q/T, where ΔS is the change in entropy, Q is the heat exchanged, and T is the absolute temperature.
- Third Law: As temperature approaches absolute zero, the entropy of a perfect crystal approaches a constant minimum.
How Do Equations in Thermodynamics Describe Energy Transfers?
Equations in thermodynamics are crucial for describing how energy is transferred within a system or between systems. The most common processes include:
- Isothermal Process: A process that occurs at a constant temperature. The equation used is Q = W, indicating that the heat added to the system is equal to the work done by the system.
- Adiabatic Process: A process in which no heat is exchanged with the surroundings. The equation is ΔU = W, meaning that the change in internal energy is equal to the work done on or by the system.
- Isochoric Process: A process that occurs at constant volume. Here, the equation is Q = ΔU, indicating that the heat added to the system results in a change in internal energy.
- Isobaric Process: A process at constant pressure, described by the equation Q = ΔU + PΔV, where P is pressure and ΔV is the change in volume.
What Role Do State Functions Play in Thermodynamics?
State functions are properties that depend only on the state of a system and not on how it reached that state. Common state functions in thermodynamics include:
- Internal Energy (U): The total energy contained within a system.
- Enthalpy (H): The total heat content of a system, defined as H = U + PV, where P is pressure and V is volume.
- Entropy (S): A measure of the disorder or randomness in a system.
- Gibbs Free Energy (G): The energy available to do work at constant temperature and pressure, given by G = H - TS, where T is temperature and S is entropy.
How Are Equations in Thermodynamics Applied in Real Life?
The equations in thermodynamics have numerous practical applications across various fields. Here are some examples:
- Engineering: Thermodynamic principles are applied in the design of engines, refrigerators, and HVAC systems to optimize energy efficiency.
- Chemistry: Understanding reaction thermodynamics allows chemists to predict the outcomes of chemical reactions and the energy changes involved.
- Environmental Science: Thermodynamic equations help in analyzing energy flows in ecosystems and the impact of human activities on energy resources.
- Aerospace: Engineers use thermodynamics to analyze the performance of aircraft and rockets, optimizing thrust and fuel efficiency.
What Are Some Common Equations in Thermodynamics?
Several key equations are commonly used in thermodynamics, including:
- Ideal Gas Law: PV = nRT, where P is pressure, V is volume, n is the number of moles, R is the ideal gas constant, and T is temperature.
- Clausius Inequality: dQ ≤ TdS, which states that the change in heat is less than or equal to the product of temperature and change in entropy.
- Maxwell Relations: A set of equations that relate different thermodynamic potentials, derived from the second derivatives of state functions.
- Heat Capacity Equations: C = ΔQ/ΔT, where C is heat capacity, ΔQ is the heat added, and ΔT is the temperature change.
How Can One Master the Equations in Thermodynamics?
Mastering the equations in thermodynamics requires a combination of theoretical understanding and practical application. Here are some tips to help:
- Study the Fundamentals: Ensure a solid grasp of basic concepts such as energy, work, heat, and temperature.
- Practice Problem-Solving: Regularly engage in solving thermodynamic problems to apply the equations and reinforce your understanding.
- Utilize Visual Aids: Diagrams, graphs, and charts can help visualize concepts and relationships between variables.
- Collaborate with Peers: Discussing concepts with fellow students or professionals can provide new insights and deepen your understanding.
What Future Developments Can We Expect in Thermodynamics?
The field of thermodynamics continues to evolve, with ongoing research aimed at improving energy efficiency and sustainability. Future developments may include:
- Advanced Materials: Research into new materials that enhance thermodynamic efficiency in energy systems.
- Renewable Energy Technologies: Innovations in harnessing and converting renewable energy sources will rely heavily on thermodynamic principles.
- Quantum Thermodynamics: Exploring the thermodynamic behavior of systems at the quantum level may yield new insights into energy transfer and transformation.
- Thermodynamics in Biological Systems: Understanding how living organisms manage energy flows can lead to breakthroughs in medicine and biotechnology.
In conclusion, equations in thermodynamics form the backbone of understanding energy interactions in systems. By grasping these equations and their implications, one can unlock the potential to innovate and improve energy systems across various fields. The study of thermodynamics is not just about equations; it is about comprehending the intricate dance of energy that shapes our world.
Article Recommendations
- Reflex Compound Bow
- Ap Precalculus Unit 3 Review
- Quality Metal Detectors
- Driving Test Edmond Ok
- Bi Fold Exterior Patio Doors
- Elements Compounds And Mixtures Answer Key
- Motion Ai Vs
- Cars With Great Audio Systems
- How To Use Rabbitfx
- Robin Sharma Wife
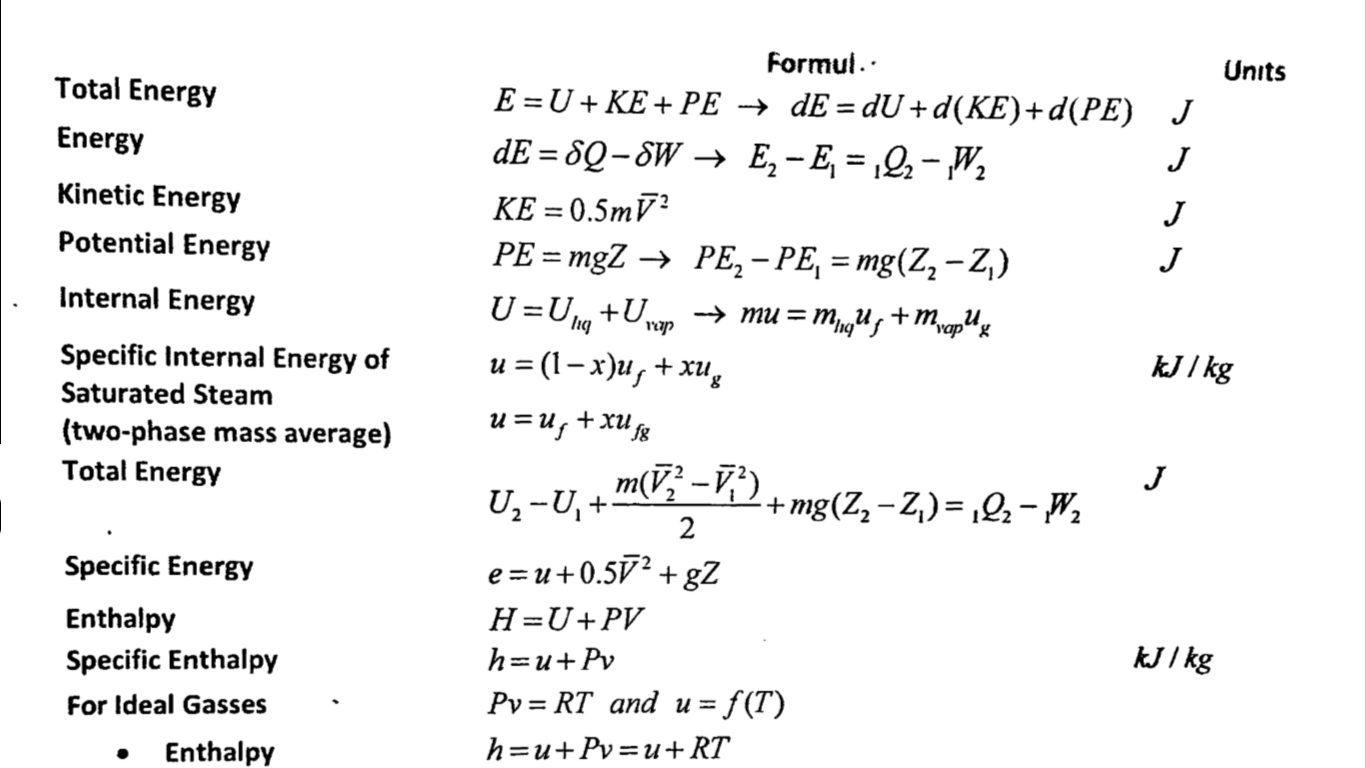

